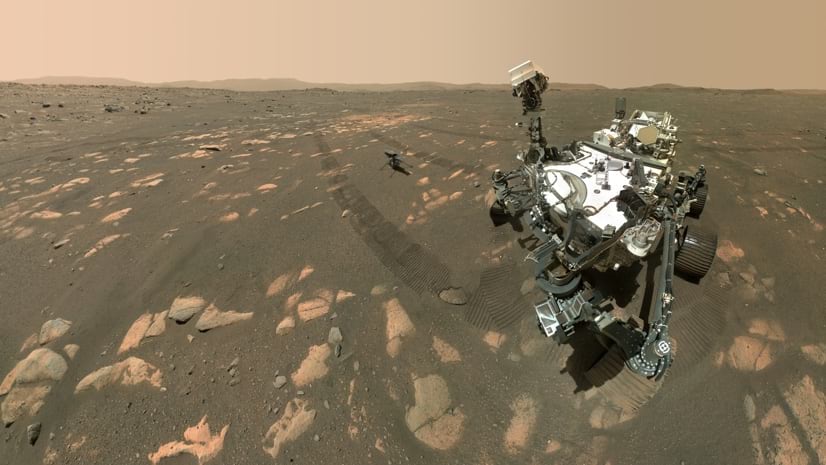
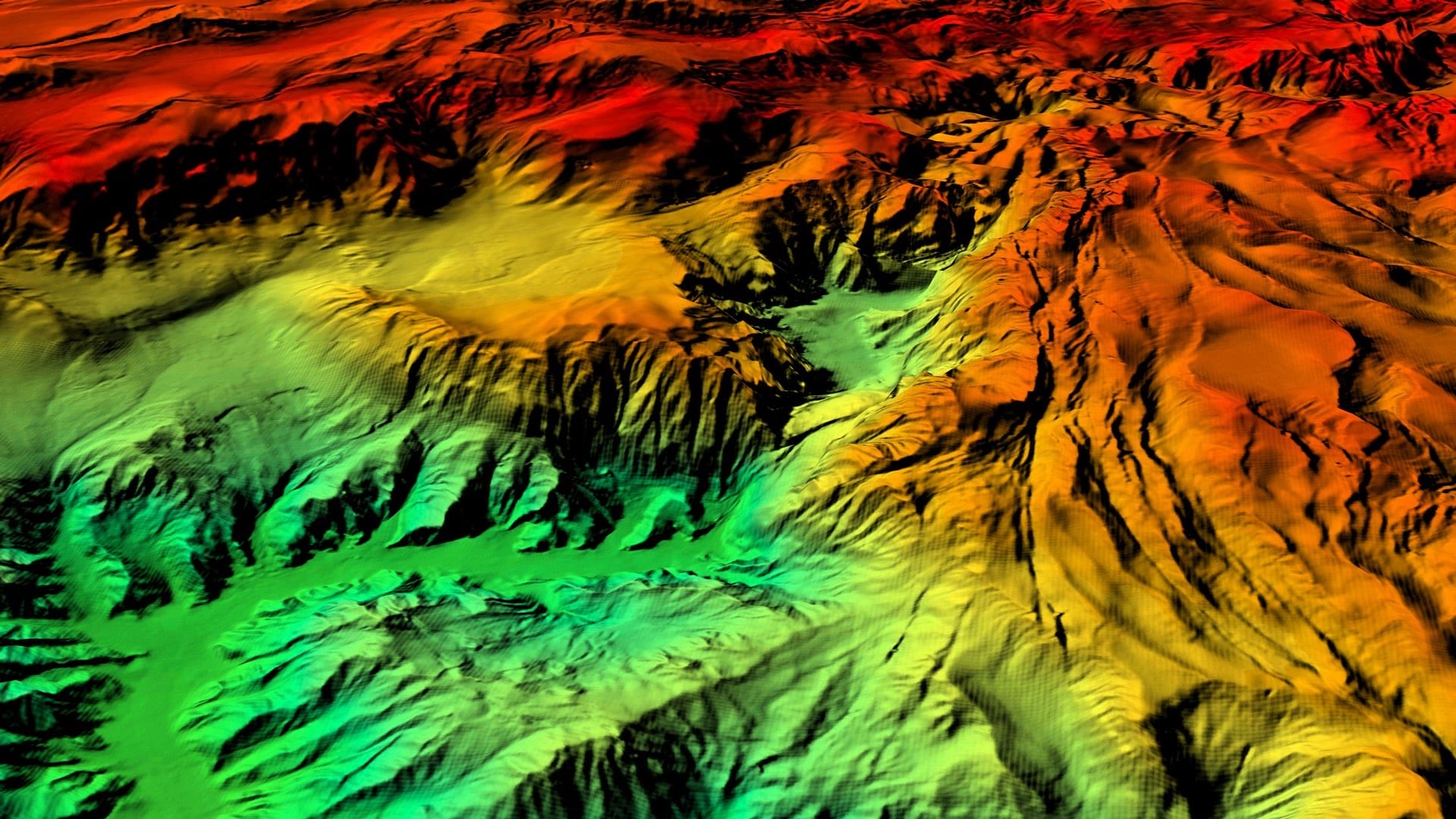
December 5, 2019 |
August 2, 2021
The successful landing of the National Aeronautics and Space Administration (NASA) Perseverance rover and accompanying Ingenuity helicopter on Mars on February 18, 2021, marked the first time a map was used to land on that planet. Certainly, maps have been instrumental during all prior Mars exploratory missions, read by people on Earth. For this Mars mission, the rover used a hazard map, created with a geographic information system (GIS), to find a safe landing spot. The Perseverance robot scanned the terrain, read its onboard map, and fired its rockets to angle over to one of the safe landing spots designated on the map.
Several teams at NASA Jet Propulsion Laboratory (JPL) built the systems that made the navigation fully autonomous. For this Mars mission, mapping reached a whole new level. The rover could look at the map while landing and then made a decision based on that map.
There has never been joystick control on any Mars landing because it can take more than 20 minutes for radio communication to travel from Earth to Mars—a distance of 130 million miles. To solve this dilemma in previous missions, boulder-free landing sites over tens of kilometers in diameter were preselected. While this approach has achieved safe landings, the strategy put prior rovers farther away from the more interesting rocks.
For the Mars 2020 mission, the team chose the Jezero crater because it was geologically interesting. It looks as if water carved a valley through the crater rim and filled the crater with a lake, depositing sediments in a river delta made up of clays and various rocks clumped near the inlet. Given Jezero’s past watery environment, scientists hope the sediments and rocks contain preserved organic molecules. The long-term mission of Perseverance is to gather rock core samples that a future mission will retrieve and return to Earth to determine if they contain the remains of life.
The promising Jezero crater site had been mapped extensively prior to this landing. Now that the craft have landed on Mars’s surface and begun exploration, mapping has increased in even greater detail. New maps are created every day, adding to the context about where the rover is, and GIS records where science is happening.
A year before the landing, the Mars 2020 team members began mapping the landing site’s geology using ArcGIS Pro, and about six months prior they started to map exciting places for the rover to go. Details are gathered in layers in the GIS using the same high-resolution basemaps that helped guide the rover to a safe landing.
The team relies on the ability of GIS to scale to any level, from the global to microscopic mineralogy. The ability to combine layers at different scales lets the team vertically integrate scientific information.
In the past, NASA’s Mars teams used different maps: one for the rover team to guide the rover, and one for the science team to find interesting areas to explore.
Now, the teams operate from one basemap that multiple partners contribute to, including the country’s mapping authority, the US Geological Survey (USGS). The USGS Astrogeology Mapping, Remote-sensing, Cartography, Technology, and Research (MRCTR, pronounced “Mercator”) GIS Lab takes raw images and derives information in the form of GIS layers that everyone can explore.
With all the scientific inputs, the map continues to be improved and has become a common language between teams.
There is a natural tug between scientists and rover drivers because the geologists want to go anywhere there are interesting rocks, and the drivers are focused on safety. The map plays a key communications role between the two, to discuss samples of scientific interest and mobility constraints.
Mapping any planet benefits from lessons learned on Earth. It starts with defining the shape and radius of the planet, then the spin axis to determine the poles. From there, a latitude and longitude system are developed and Cartesian coordinates of x, y, and z are applied. All the tools we use to map the Earth work the same to map other planets.
In 1996, NASA launched the Mars Global Surveyor, which has many sensors on board—including the Mars Orbiter Camera that captures a daily global map of Mars—primarily for the purpose of looking at weather patterns. The Mars Orbital Laser Altimeter (MOLA) provides global topography to understand exactly where things are on the surface in three-dimensional space, defining the coordinate system for latitude and longitude on Mars.
In 2005, NASA launched the Mars Reconnaissance Orbiter, with sensors that captured images that now form a new Mars basemap for GIS work. The Context Camera (CTX) on that craft provides big-picture images that span 25 miles across at six meters per pixel resolution. In 2017, the craft had captured enough images to have essentially global coverage, providing a new global basemap. The High Resolution Imaging Science Experiment (HiRISE) camera on the same orbiter captures imagery at 25 centimeters per pixel resolution, which is as fine-grained as the publicly accessible satellite imagery of Earth. At this resolution, rover wheel tracks are visible on the planet’s surface. The sensor focuses on areas of particular interest and has captured roughly 5 percent of the surface of Mars at high resolution.
All imagery is layered to build an image pyramid, with finer resolution at the top and a documented tree of where these images are relative to each other.
The areas captured at highest resolution are the areas of greatest interest, including the Jezero crater where the Mars 2020 mission landed. From high-resolution imagery, the team derived a digital elevation model, which allowed engineers to see rocks and high slopes and assess landing sites.
For more than four years prior to the Mars 2020 mission, a hazard mapping team at JPL conducted the painstaking work of identifying and mapping places to avoid around every site of interest.
The work began when there were 16 possible landing sites. Each were evaluated for safety and scientific value, and the understanding and resolution of the data grew finer grained as the sites were whittled down to 8 and then 4 sites, and finally 1.
Terrain hazards on Mars take many shapes. Boulders are dangerous obstacles. Cliffs obviously can’t be climbed, and the rocks at the bottom of cliffs point to the potential dangers. There are also sandy patches where the rover might slip or get stuck. And the rover can’t go up steep terrain.
The images from different angles captured by the HiRISE sensor gave the team the ability to create a three-dimensional slope map and the relative risk associated with them. The mapping team looked at the surface images to see such things as sand ripples to avoid places the rover might get stuck. Hours of manual effort pouring over the images went into making the hazard map of the potentially inescapable regions within the landing ellipse.
Many considerations went into determining go and no-go landing sites. Steep terrain obviously is a problem, as are craters, as it’s harder to drive up out of a hole than down off a hill.
Boulders are another consideration, and scientists have developed an automated rock detection algorithm to determine the size of each rock based on its shadow and to mark off any areas with rocks higher than the rover belly.
The Jezero site it rockier and has more hazards than past landing sites. This tricky spot was possible thanks to the TRN system and the hazard map. The landing site proved ideal, without any large rocks or deep sand.
Traveling at a speed of two and a half centimeters per second and covering 200 meters (656 feet) per day, it takes the rover time to explore. The rover can drive by itself by taking pictures with its stereo cameras that allow it to sense depth, construct topography, and see hazards.
Instead of a map of the whole region, the rover keeps track of its location and generates its own maps onboard to plan local routes. The broader map is maintained on Earth.
Now, the Ingenuity helicopter can scout ahead to give the rover team additional insights. The ground crew can send a point in space where they want the rover to go, and it figures out how to get there. For the helicopter, flight paths are mapped and then it executes the preplanned trajectory. Both are equipped with cameras to take pictures along the way that are added to the map.
The images the rover and the helicopter take get added to GIS to inform strategic planning. Every day, ArcGIS Pro is used to make new maps that are handed to the drivers and pilots to plan where to go next.
GIS helps the mapping team see the data they have and see where they may want to go. It also lets them plan how to safely and efficiently get there.
The Mars Helicopter Ingenuity team used the same maps and higher resolution images taken by the rover to plan where to deploy it and conduct its first three flights. Then, the Ingenuity helicopter transitioned to becoming a scout for the rover, and mapping took on a whole new operational role. The helicopter expanded the team’s awareness in areas they haven’t driven yet. The helicopter provides top-down detail and context of what’s ahead. It can go places the rover can’t and explore from a different vantage point.
The simple Ingenuity drone craft has a pair of 1.2-meter carbon fiber rotors, a body about the size of a tissue box, and four thin legs. It proved early in the mission that flight is possible, and it continues to add more value beyond its simple technology demonstration mission.
Flight alone was a huge accomplishment, given the thin atmosphere on Mars, the power requirements of flight, and communications challenges.
During the early flights, dust on the solar panels was blown off and the battery is charging well. Communication with the rover is better than expected, which makes flight further away from the rover a possibility. And the landing gear, that the crew worried would wear, hasn’t seen any damage because landings have been gentle.
The helicopter has a downward-facing VGA camera used for feature tracking to figure out relative position, speed, and direction, which is how the helicopter navigates along the planned path. A 13-megapixel color camera has been used to survey new areas and send back pictures that contribute scouting and imaging to support the scientific exploration.
For flight four, a 270-meter round trip to the south and back, the helicopter flew over an area the rover hadn’t been yet. With the fresh images, the team was able to assess the terrain and choose the next safest place to land.
Perseverance has cameras that can get stereo images to derive 3D terrain meshes, sometimes extending over 100 feet, but it can’t see behind rocks like the helicopter can. For now, the helicopter is tethered because it needs the rover to speak to Earth. Future helicopters are planned with more autonomy to take a further afield approach to tag team exploration.
A key goal for Perseverance is to collect a returnable cache of soil and rock samples. The rover can drill into rocks; extract a rock core that’s about the size of a dry-erase marker; then put that into a tube; seal it; and drop it on the surface for a future return-to-Earth mission.
The samples will be collected from many different terrains and most importantly, precisely geographically referenced. The goal is to collect a broad diversity of rock samples that tell a story about the geologic process or that may have a potential biosignature as a sign of life.
To date, there aren’t any samples from a known location on Mars. The meteorites linked to Mars don’t have a known location. So the idea is to collect samples and build a story about the context from the sample site, its broader outcrop unit, its geologic unit, and the regional geology. Knowledge of the rocks and their history will allow scientists to understand other like areas of Mars and to potentially piece together the evolution of the planet.
Looking at the images lets the scientists see boundaries between different rock types, and if they represent different layers. There are a host of questions about how the layers formed and how the planet transformed.
The Mars Sample Return project (MSR) is being coordinated with the European Space Agency, which will support the effort to build the fetch rover and return-to-Earth orbiter used to return the samples. To get the broadest possible diversity of samples, geologic maps are key to communication between teams. Sample site maps are constantly evolving to guide the fetch mission slated for the end of this decade.
Maps provide context for the rover, the helicopter, the return sample mission, and for related scientific inquiries.
The Curiosity rover mission in 2012 was a proof point for the power of the maps. From its landing site, the rover was supposed to have driven due south but instead was rerouted after the team consulted the latest map that showed three geologic units coming together just 300 meters away. The lake sediments in that spot answered major mission goals within a year, instead of two, and helped validate the value of the mapping team.
For the Mars 2020 mission, the map shows a lot more detail about the Jezero crater, and many other newly mapped sites are of interest to the team. Again, the maps are leading the way.
December 5, 2019 |
April 22, 2020 |
February 16, 2021 |